The two different types of rotations described are:
- Simply rotat...
Click here to see more
Here is an example of a rigid rotation as described in the paper:
...
The researchers used "mirror image" objects for the different pairs...
The main observation from these graphs is that the reaction times a...
The effect described in this paper does not seem to depend specific...
Mental rotation experiments like this are almost unique amongst ima...
This findings in this study went against the then popular Behaviori...
A. Michael Noll is an American engineer and actually a very early ...
Some researchers would go on to propose alternative explanations to...
solution
is
shown
for
day
1
only
(16),
because,
even
though
the
intake
was
relatively
small
(mean
intake,
98
ml),
the
cats
became
ill.
Two
drank
sucrose
almost
continuously
up
to
the
criterion
and
subsequently
vomited
and
devel-
oped
diarrhea.
The
others
did
not
vomit
but
developed
diarrhea.
The
ill-
ness
apparently
iled
to
conditioned
aversion.
After
a
week's
rest,
cats
re-
jected
0.375M
sucrose
(mean
intake,
18
ml).
This
same
thing
happened
with
O.5M
sucrose
solution
and
24-hour
in-
take.
Frings's
(9)
finding
that
sucrose
in
dilute
milk
(one
part
milk
to
four
parts
water)
is
preferred
by
cats
fits
in
well
with
the
result
presented
here.
Mean
sodium
and
chlorine
content
for
whole
milk
so
diluted
would
approximate
0.006M
NaCl
(17).
The
exact
whole-
mouth
salivary
NaCl
concentration
for
the
cat
is
not
known,
but
it
must
fall
between
0.01M
and
0.16M
NaCl
(18).
For
adapting
concentrations
in
this
range,
electrophysiological
data
(3)
suggest
that
the
0.006M
NaCl
in
the
milk
used
by
Frings
would
at
least
partially
suppress
the
water-after-NaCl
response.
The
taste
of
water
has
been
widely
ignored
in
behavioral
testing.
It
is
now
clear
that
water
should
be
regarded
not
as
a
neutral
solvent
but
rather
asa
taste
stimulus
itself.
The
implications
for
receptor
mechanisms
are
still
un-
clear.
Water
appears
to
produce
some
responses
by
removing
other
stimuli
(2),
but
it
may
also
stimulate
recep-
tors
directly
[see
(19)
for
a
review
of
various
structural
models
of
water].
Nevertheless,
electrophysiological
stud-
ies
can
suggest
how
water
tastes
can
be
manipulated
to
assess
the
taste
of
any
given
substance
in
water.
L.
M.
BARTOSHUK
John
B.
Pierce
Foundation
Laboratory,
290
Congress
Avenue,
New
Haven,
Connecticut
06519
M.
A.
HARNED
L.
H.
PARKS
Pioneering
Research
Laboratory,
U.S.
Army
Natick
Laboratories,
Natick,
Massachusetts
01760
References
and
Notes
1.
G.
Liljestrand
andY.
Zotterman,
Acta
Physiol.
Scand.
32,
291
(1954);
Y.
Zotterman,
ibid.
37,
60
(1956);
-
and
H.
Diamant,
Nature
183,
191
(1959).
2.
M.
J.
Cohen,
S.
Hagiwara,
Y.
Zotterman,
Acta
Physiol.
Scand.
33,
316
(1955).
3.
L.
M.
Bartoshuk,
thesis,
Brown
University
(1965).
4.
and
C.
Pfaffmann,
Fed.
Proc.
24,
207
(1965);
C.
Pfaffmann,
in
Olfaction
and
Taste
C.
Pfaffmann,
Ed.
(Rockefeller
Univ.
Press,
New
York,
1969),
vol.
3,
p.
528.
19
FEBRUARY
1971
5.
L.
M.
Bartoshuk,
D.
H.
McBumey,
C.
Pfaff-
mann,
Science
143,
967
(1964);
L.
M.
Barto-
shuk,
Percept.
Psychophys.
3,
69
(1968);
D.
H.
McBurney,
J.
Exp.
Psychol.
72,
869
(1966);
in
Olfaction
and
Taste,
C.
Pfaffmann,
Ed.
(Rockefeller
Univ.
Press,
New
York,
1969),
vol.
3,
p.
407.
6.
0.
Maller
and
M.
R.
Kare,
Anim.
Behav.
15,
8
(1967);
V.
G.
Dethier
and
M.
V.
Rhoades,
J.
Exp.
Zool.
126,
177
(1954);
C.
Duncan,
Anim.
Behav.
8,
54
(1960);
H.
L.
Jacobs
and
M.
L.
Scott,
Poultry
Sci.
36,
8
(1957);
B.
P.
Halpern,
Amer.
J.
Physiol.
203,
541
(1962);
M.
R.
Kare
and
M.
S.
Ficken,
in
Olfaction
and
Taste,
Y.
Zotterman,
Ed.
(Pergamon,
New
York,
1963),
vol.
2,
p.
285;
J.
Ganchrow
and
G.
L.
Fisher,
Psychol.
Rep.
22,
503
(1968);
C.
Pfaffmann,
Amer.
Psychol.
20,
21
(1965).
7.
J.
A.
Carpenter,
J.
Comp.
Physiol.
Psychol.
49,
139
(1956).
8.
Y.
Zotterman,
Skand.
Archiv
Physiol.
72,
73
(1935);
C.
Pfaffmann,
J.
Cell.
Comp.
Physiol.
17,
243
(1941).
9.
H.
Frings,
Experientia
(Basel)
7,
424
(1951).
10.
C.
Pfaffmann,
J.
Neurophysiol.
18,
492
(1955).
11.
The
sensitivities
to
NaCl,
QHCI,
sucrose,
and
HCI
appear
to
be
independently
associated
in
accordance
with
the
random
distribution
hy-
pothesis
of
M.
Frank
and
C.
Pfaffmann
[Sci-
ence
164,
1183
(1969)].
In
addition,
the
four
contingencies
producing
water
responses
also
appear
to
be
independently
associated
in
ac-
cordance
with
this
hypothesis.
12.
The
correlation
coefficient
for
water-after-
NaCl
responses
and
NaCl
responses
was
-0.83
(P
<
.005).
Only
those
fibers
were
in-
cluded
for
which
at
least
one
response
met
the
criterion.
Previous
reports
of
a
negative
correlation
between
water
responses
and
NaCI
responses
[Cohen
et
al.
(2);
J.
Nagaki,
S.
Yamashita,
M.
Sato,
Jap.
J.
Physiol.
14,
67
(1964)]
probably
reflect
the
negative
correla-
tion
between
water-after-NaCl
and
NaCl
re-
sponses
since
the
rinse
was
Ringer
solution.
The
correlation
coefficients
for
NaCI
and
water-after-QHCl,
water-after-sucrose,
and
water-after-HCI
are
-0.65
(P
<
.05),
-0.54
(P
>
.05),
and
-0.28
(P
>
.2),
respectively.
Human
subjects
are
often
able
to
determine
that
two
two-dimensional
pictures
portray
objects
of
the
same
three-dimensional
shape
even
though
the
objects
are
depicted
in
very
different
orientations.
The
experiment
reported
here
was
designed
to
measure
the
time
that
subjects
require
to
determine
such
identity
of
shape
as
a
function
of
the
angular
difference
in
the
portrayed
ori-
entations
of
the
two
three-dimensional
objects.
This
angular
difference
was
produced
either
by
a
rigid
rotation
of
one
of
two
identical
pictures
in
its
own
picture
plane
or
by
a
much
more
complex,
nonrigid
transformation,
of
one
of
the
pictures,
that
corresponds
to
a
(rigid)
rotation
of
the
three-dimensional
ob-
ject
in
depth.
This
reaction
time
is
found
(i)
to
13.
We
thank
M.
Dvorak
and
0.
Starkof
the
Food
Sciences
Laboratory
at
the
Natick
Army
Laboratories
for
analyses
of
the
atomic
ab-
sorption
spectra
of
tap
and
distilled
water
samples.
Samples
were
analyzed
for
Na,
K,
Ca,
Mg,
and
halide.
Tap
water
checked
week-
ly
for
2
months
remained
relatively
constant
with
mean
levels
of
9.6
X
10-4M
Na,
6.8
X
10-5M
K,
7.1
X
10-4M
Ca,
2.2
X
10-4M
Mg,
and
1.0
X
10-3M
halide
(predominantly
Cl).
Distilled
water
contained
8.7
X
10-7M
Na,
5.1
X
10-6M
K,
2.5
X
10-6M
Ca,
and
4.1
X
10-7M
Mg.
14.
P.
Hore
and
M.
Messer,
Comp.
Biochem.
Physiol.
24,
717
(1968).
15.
S.
Siegel,
Nonparametric
Statistics
(McGraw-
Hill,
New
York,
1956).
16.
Since
testing
was
discontinued
after
1
day,
the
position
of
sucrose
was
not
counter-
balanced.
To
ensure
that
position
preference
could
not
account
forthe
results
at
a
sucrose
concentration
of
0.375M,
position
preference
under
all
other
conditions
was
tested
with
a
two-tailed
Walsh
test
(15).
The
results
were
not
significant
(P
>
.1).
17.
K.
Diem,
Ed.,
Scientific
Tables
(Geigy
Pharmaceuticals,
Ardsley,
N.Y.,
ed.
6,
1962),
p.
516.
18.
L.
H.
Schneyer
and
C.
A.
Schneyer,
in
Hand-
book
of
Physiology:
Alimnentary
Canal,
C.
F.
Code,
Ed.
(American
Physiological
Society,
Washington,
D.C.,
1967),
vol.
2.
19.
0.
Eisenberg
and
W.
Kauzmann.
The
Struc-
ture
and
Properties
of
Water
(Oxford
Univ.
Press,
New
York,
1969).
20.
Electrophysiological
data
and
preliminary
be-
havioral
data
were
obtained
at
Brown
Uni-
versity
where
work
was
supported
in
part
by
a
PHS
predoctoral
fellowship
to
L.M.B.
and
NSF
grants
G-14332
(to
C.
Pfaffmann)
and
GB-2754
(to
C.
Pfaffmannand
L.M.B.).
Final
behavioral
data
were
collected
at
the
U.S.
Army
Natick
Laboratories.
We
thank
R.
L.
Gentile,
J.
C.
Stevens,
L.
E.
Marks,
and
W.
S.
Cain
for
valuable
comments
on
the
manu-
script.
19
October
1970;
revised
13
November
1970
*
increase
linearly
with
the
angular
dif-
ference
in
portrayed
orientation
and
(ii)
to
be
no
longer
for
a
rotation
in
depth
than
for
a
rotation
merely
in
the
picture
plane.
These
findings
appear
to
place
rather
severe
constraints
on
pos-
sible
explanations
of
how
subjects
go
about
determining
identity
of
shape
of
differently
oriented
objects.
They
are,
however,
consistent
with
an
explanation
suggested
by
the
subjects
themselves.
Although
introspective
reports
must
be
interpreted
with
caution,
all
subjects
claimed
(i)
that
to
make
the
required
comparison
they
first
had
to
imagine
one
object
as
rotated
into
the
same
orientation
as
the
other
and
that
they
could
carry
out
this
"mental
rotation"
at
no
greater
than
a
certain
limiting
rate;
and
(ii)
that,
since
they
perceived
the
two-dimensional
pictures
as
objects
701
Mental
Rotation
of
Three-Dimensional
Objects
Abstract.
The
time
required
to
recognize
that
two
perspective
drawings
portray
objects
of
the
same
three-dimensional
shape
is
found
to
be
(i)
a
linearly
increasing
function
of
the
angular
difference
in
the
portrayed
orientations
of
the
two
objects
and
(ii)
no
shorter
for
differences
corresponding
simply
to
a
rigid
rotation
of
one
of
the
two-dimensional
drawings
in
its
own
picture
plane
than
for
differences
corresponding
to
a
rotation
of
the
three-dimensional
object
in
depth.
on May 26, 2012www.sciencemag.orgDownloaded from
in
three-dimensional
space,
they
could
imagine
the
rotation
around
whichever
axis
was
required
with
equal
ease.
In
the
experiment
each
of
eight
adult
subjects
was
presented
with
1600
pairs
of
perspective
line
drawings.
For
each
pair
the
.subject
was
asked
to
pull
a
right-hand
lever
as
soon
as
he
deter-
mined
that
the
two
drawings
portrayed
objects
that
were
congruent
with
respect
to
three-dimensional
shape
and
to
pull
al
lelft-hand
lever
as
soon
as
he
deter-
rniind
that
the
two
drawings
depicted
objects
of
different
three-dimensional
shapes.
According
to
a
random
se-
quence,
in
half
of
the
pairs
(the
"same"
pairs)
the
two
objects
could
be
rotated
into
congruence
with
each
other
(as
in
Fig.
1,
A
and
B).
and
in
the
other
half
(the
"different"
pairs)
the
two
objects
differed
by
a
reflection
as
well
as
a
rotation
and
could
not
be
rotated
into
congruence
(as
in
Fig.
IC).
The
choice
of
objects
that
were
mir-
ror
images
or
"isomers"
of
each
other
for
the
"different"
pairs
was
intended
to
prevent
subjects
from
discovering
Fig.
1.
Examples
of
pairs
of
perspective
line
dr-awings
presented
to
the
subjects.
(A)
A
"same"
pair,
which
differs
by
an
80'
rotation
in
the
picture
plane;
(B)
a
"same"
pair,
which
differs
by
an
80°
ro-
tation
in
depth;
and
(C)
a
"different"
pair,
wshich
cannot
be
brought
into
con-
gruence
by
aniy
r-otation.
702
some
distinctive
feature
possessed
by
only
one
of
the
two
objects
and
thereby
reachir;g
a
decision
of
noncongruence
without
actually
having
to
carry
out
any
mental
rotation.
As
a
further
pre-
caution,
the
ten
different
three-dimen-
sional
objects
depicted
in
the
various
perspective
drawings
were
chosen
to
be
relatively
unfamiliar
and
meaning-
less
in
overall
three-dimensional
shape.
Each
object
consisted
of
ten
solid
cubes
attached
face-to-face
to
form
a
rigid
armlike
structure
with
exactly
three
right-angled
"elbows"
(see
Fig.
1).
The
set
of
all
ten
shapes
included
two
subsets
of
five:
within
either
subset,
no
shape
could
be
transformed
into
itself
or
any
other
by
any
reflection
or
rota-
tion
(short
of
360°).
However,
each
shape
in
either
subset
was
the
mirror
image
of
one
shape
in
the
other
sub-
set,
as
required
for
the
construction
of
the
"different"
pairs.
For
each
of
the
ten
objects,
1
8
dif-
ferent
perspective
projections
corre-
sponding
to
one
complete
turn
around
the
vertical
axis
by
20°
steps-were
generated
by
digital
computer
and
asso-
ciated
graphical
output
(1).
Seven
of
the
18
perspective
views
of
each
object
were
then
selected
so
as
(i)
to
avoid
any
views
in
which
some
p.art
of
the
object
was
wholly
occluded
by
another
part
and
yet
(ii)
to
permit
the
construction
of
two
pairs
that
differed
in
orientation
by
each
possible
angle,
in
20°
steps,
from
00
to
180°.
These
70
line
draw-
ings
were
then
reproduced
by
photo-
offset
process
and
were
attached
to
cards
in
pairs
for
presentation
to
the
subjects.
Half
of
the
"same"
pairs
(the
"depth"
pairs)
represented
two
objects
that
dif-
fered
by
some
multiple
of
a
20°
rota-
tion
about
a
vertical
axis
(Fig.
1
B).
For
each
of
these
pairs,
copies
of
two
appro-
priately
different
perspective
views
were
simply
attached
to
the
cards
in
the
orientation
in
which
they
were
origi-
nally
gener.ated.
The
other
half
of
the
"'same"
pairs
(the
"picture-plane"
pairs)
represented
two
objects
that
differed
by
some
multiple
of
a
200
rotation
in
the
plane
of
the
drawings
themselves
(Fig.
lA).
For
each
of
these,
one
of
the
seven
perspective
views
was
selected
for
each
object
and
two
copies
of
this
picture
were
attached
to
the
card
in
appropriately
different
orientations.
Al-
together,
the
1600
pairs
presented
to
each
subject
included
800
"same"
pairs,
which
consisted
of
400
unique
pairs
(20
"depth"
and
20
"picture-plane"
pairs
at
each
of
the
ten
angular
differences
from
0°
to
1800),
each
of
which
was
presented
twice.
The
remaining
800
p.airs,
randomly
intermixed
with
these,
consisted
of
400
unique
"different"
pairs,
each
of
which
(again)
was
pre-
sented
twice.
Each
of
these
"different"
pairs
corresponded
to
one
"same"
pair
(ot
either
the
"depth"
or
"picture-plane"
valriety)
in
which,
however,
one
of
the
three-dimensional
objects
had
been
re-
flected
about
some
plane
in
three-di-
men.isonal
space.
Thus
the
two
objects
in
each
"different"
pair
differed,
in
gen-
eral,
by
both
a
reflection
and
a
rota-
tion.
The
1600
pairs
were
grouped
into
blocks
of
not
more
than
200
and
pre-
sented
over
eight
to
ten
1-hour
ses-
sions
(depending
upon
the
subject).
Also,
although
it
is
only
of
incidental
interest
here,
each
such
block
of
pres-
entaltions
was
either
"pure,"
in
that
all
pairs
involved
rotations
of
the
same
type
("depth"
or
"picture-plane"),
or
"mixed,"
in
that
the
two
types
of
rot.a-
5-
A
(Picture-plane
pairs)
T
4
0.
2
_
a)
E
in
,
,
,
,
.
.
0
20
40
60
80
100
120
140
160
180
0
B
(Depth
pairs)
E
.2
4
i~~~~~~)
~~~~~T
13
2
0
20
40
60
80
100
120
140
160
180
Angle
of
rotation
(degrees)
Fig.
2.
Mlean
reaction
times
to
two
perspec-
tive
line
drawings
poitraying
objects
of
the
s,ame
three-dimensional
shape.
Times
are
plotted
as
a
function
of
angular
difference
in
poitr-ayed
orientation:
(A)
for
pairs
differing
by
a
rotation
in
the
pictu:-e
plane
only:
and
(B)
for
pairs
differing
by
a
rotation
in
depth.
(The
centers
of
the
circles
indicate
the
means
and,
when
they
extend
far
enough
to
show
outside
these
circles,
the
vertical
bars
around
each
circle
indicate
a
conservative
esti-
mate
of
the
standard
error
of
that
mean
based
on
the
distribution
of
the
eight
component
means
contributed
by
the
in-
dividtual
subjects.)
SCIENCE,
VOL.
171
on May 26, 2012www.sciencemag.orgDownloaded from
tion
were
randomly
intermixed
within
the
same
block.
Each
trial
began
with
a
warning
tone,
which
was
followed
half
a
second
later
by
the
presentation
of
a
stimulus
pair
and
the
simultaneous
onset
of
a
timer.
The
lever-pulling
response
stopped
the
timer,
recorded
the
subject's
reaction
time
and
terminated
the
visual
display.
The
line
drawings,
which
averaged
be-
tween
4
and
5
cm
In
maximum
linear
extent,
appeared
at
a
viewing
distance
of
about
60
cm.
They
were
positioned,
with
a
center-to-center
spacing
that
subtended
a
visual
angle
of
90,
in
two
circular
apertures
in
a
vertical
black
surface
(see
Fig.
1,
A
to
C).
The
subjects
were
instructed
to
re-
spond
-as
quickly
as
possible
while
keep-
ing
errors
to
a
minimum.
On
the
aver-
age
only
3.2
percent
of
the
responses
were
incorrect
(ranging
from
0.6
to
5.7
percent
for
individual
subjects).
The
reaction-time
data
presented
below
in-
clude
only
the
96.8
percent
correct
re-
sponses.
However,
the
data
for
the
in-
correct
responses
exhibit
a
similar
pat-
tern.
In
Fig.
2,
the
overall
means
of
the
reaction
times
as
a
function
of
angular
difference
in
orientation
for
all
correct
(right-hand)
responses
to
"same"
pairs
are
plotted
separately
for
the
pairs
dif-
fering
by
a
rotation
in
the
picture
plane
(Fig.
2A)
and
for
the
pairs
differing
by
a
rotation
in
depth
(Fig.
2B).
In
both
cases,
reaction
time
is
a
strikingly
linear
function
of
the
angular
difference
be-
tween
the
two
three-dimensional
objects
portrayed.
The
mean
reaction
times
for
individual
subjects
increased
from
a
value
of
about
1
second
at
00
of
rota-
tion
for
all
subjects
to
values
ranging
from
4
to
6
seconds
at
1800
of
rotation,
depending
upon
the
particular
individ-
ual.
Moreover,
despite
such
variations
in
slope,
the
linearity
of
the
function
is
clearly
evident
when
the
data
are
plotted
separately
for
individual
three-
dimensional
objects
or
for
individual
subjects.
Polynomial
regression
lines
were
computed
separately
for
each
sub-
ject
under
each
type
of
rotation.
In
all
16
cases
the
functions
were
found
to
have
a
highly
significant
linear
com-
ponent
(P
<.001)
when
tested
against
deviations
from
linearity.
No
significant
quadratic
or
higher-order
effects
were
found
(P
>
.05,
in
all
cases).
The
angle
through
which
different
three-dimensional
shapes
must
be
ro-
tated
to
achieve
congruence
is
not,
of
course,
defined.
Therefore,
a
function
like
those
plotted
in
Fig.
2
cannot
be
constructed
in
any
straightforward
man-
19
FEBRUARY
1971
ner
for
the
"different"
pairs.
The
overall
mean
reaction
time
for
these
pairs
was
found,
however,
to
be
3.8
seconds-
nearly
a
second
longer
than
the
cor-
responding
overall
means
for
the
"same"
pairs.
(In
the
postexperimental
inter-
view,
the
subjects
typically
reported
that
they
attempted
to
rotate
one
end
of
one
object
into
congruence
with
the
corresponding
end
of
the
other
object;
they
discovered
that
the
two
objects
were
different
when,
after
this
"rota-
tion,"
the
two
free
ends
still
remained
noncongruent.)
Not
only
are
the
two
functions
shown
in
Fig.
2
both
linear
but
they
are
very
similar
to
each
other
with
respect
to
intercept
and
slope.
Indeed,
for
the
larger
angular
differences
the
reaction
times
were,
if
anything,
somewhat
shorter
for
rotation
in
depth
than
for
rotation
in
the
picture
plane.
However,
since
this
small
difference
is
either
ab-
sent
or
reversed
in
four
of
the
eight
subjects,
it
is
of
doubtful
significance.
The
determination
of
identity
of
shape
may
therefore
be
based,
in
both
cases,
upon
a
process
of
the
same
general
kind.
If
we
can
describe
this
process
as
some
sort
of
"mental
rotation
in
three-
dimensional
space,"
then
the
slope
of
the
obtained
functions
indicates
that
the
average
rate
at
which
these
particu-
lar
objects
can
be
thus
"rotated"
is
roughly
600
per
second.
Of
course
the
plotted
reaction
times
necessarily
include
any
times
taken
by
the
subjects
to
decide
how
to
process
Although
it
is
now
firmly
established
that
the
hypothalamus
is
intimately
in-
volved
in
the
elaboration
of
aggressive
behavior
(1),
very
little
is
known
about
the
neural
pathways
through
which
such
behavior
is
mediated.
In
an
attempt
to
trace
out
the
circuits
which
may
be
associated
with
a
cat's
attack
upon
a
rat
we
have
employed
neuroanatomic
techniques
in
conjunction
with
stimu-
lation
experiments.
The
development
of
silver
stains
the
pictures
in
each
presented
pair
as
well
as
the
time
taken
actually
to
carry
out
the
process,
once
it
was
chosen.
However,
even
for
these
highly
prac-
ticed
subjects,
the
reaction
times
were
still
linear
and
were
no
more
than
20
percent
lower
in
the
"pure"
blocks
of
presentations
(in
which
the
subjects
knew
both
the
axis
and
the
direction
of
the
required
rotation
in
advance
of
each
presentation)
than
in
the
"mixed"
blocks
(in
which
the
axis
of
rotation
was
unpredictable).
Tentatively,
this
suggests
that
80
percent
of
a
typical
one
of
these
reaction
times
may
rep-
resent
some
such
process
as
"mental
rotation"
itself,
rather
than
a
prelimi-
nary
process
of
preparation
or
search.
Nevertheless,
in
further
research
now
underway,
we
are
seeking
clarification
of
this
point
and
others.
ROGER
N.
SHEPARD
JACQUELINE
METZLER
Department
of
Psychology,
Stanford
University,
Stanford,
California
94305
References
and
Notes
1.
Mrs.
Jih-Jie
Chang
of
the
Bell
Telephone
Lab-
oratories
generated
the
180
perspective
projec-
tions
for
us
by
means
of
the
Bell
Laboratories'
Stromberg-Carlson
4020
microfilm
recorder
and
the
computer
program
for
constructing
such
projections
developed
there
by
A.
M.
Noll.
See,
for
example,
A.
M.
Noll,
Computers
Automation
14,
20
(1965).
2.
We
thank
Mrs.
Chang
[see
(I)];
and
we
also
thank
Dr.
J.
D.
Elashoff
for
her
suggestions
concerning
the
statistical
analyses.
Assistance
in
the
computer
graphics
was
provided
by
the
Bell
Telephone
Laboratories.
Supported
by
NSF
grant
GS-2283
to
R.N.S.
9
March
1970;
revised
8
September
1970
a
capable
of
selectively
impregnating
degenerating
axoplasm
by
Nauta
(2)
has
dramatically
increased
the
ability
of
neuroanatomists
to
determine
the
po-
larity
of
conduction
and
the
areas
of
termination
of
finely
myelinated
and
unmyelinated
fiber
systems.
The
first
step
in
tracing
out
degeneration
by
this
technique
consists
in
destroying
a
small
amount
of
neural
tissue
in
a
selected
anatomic
target
area
and
then
per-
mitting
the
animal
to
survive
for
a
703
Neural
Pathways
Associated
with
Hypothalamically
Elicited
Attack
Behavior
in
Cats
Abstract.
Small
electrolytic
lesions
were
made
in
cats
through
electrodes,
which,
when
stimulated,
elicited
either
quiet
biting
attack
or
affective
paw
strike
attack
upon
rats.
The
Nauta
method
for
impregnating
degenerating
axoplasm
was
used
to
reveal
that
degeneration
resulting
from
lesions
at
quiet
attack
sites
followed
largely
along
the
course
of
the
medial
forebrain
bundle,
while
the
degeneration
after
lesions
of
affective
attack
sites
was
concentrated
more
heavily
in
the
periventricular
system.
on May 26, 2012www.sciencemag.orgDownloaded from
Discussion
The researchers used "mirror image" objects for the different pairs to make sure that the subjects were forced to mentally rotate the objects instead of trying to spot some other difference in the two images.
Some researchers would go on to propose alternative explanations to the fact that the average reaction time increases with the rotation angle.
For example, in 1976, researchers [Marcel Adam Just & Patricia Carpenter ](https://link.springer.com/article/10.3758/BF03201761) tracked subjects' eye movements whilst they did a version of the Shepard & Metzler task. They argued that the linear increase in reaction time arose not from the inner rotation of an image, but from a need to make more eye movements between the two pictures (in order to compare their features) the more they were rotated relative to one another.
This findings in this study went against the then popular Behaviorist doctrine which claimed that thought processes depended entirely upon language. With this study Shepard and Metzler showed that analog mental representations have an important role to play in thinking.
The two different types of rotations described are:
- Simply rotating the 2d drawing of the object - as if you rotate the piece of paper where the drawing was printed
- Rotating the represented object in 3d space
Here is an example of a rigid rotation as described in the paper:

Mental rotation experiments like this are almost unique amongst imagery experiments in that they depend neither on verbal reports from the subjects, nor on explicit verbal instructions to use imagery in performing the experimental task. Therefore they can be adapted for use with animal subjects, raising the possibility of providing direct evidence for the occurrence of imagery in non-human animals.
The main observation from these graphs is that the reaction times are proportional to the rotational difference. This linear relationship implies that "mental rotation" occurs at a constant speed.
The effect described in this paper does not seem to depend specifically upon **visual** imagery. Carpenter & Eisenberg found the same effect in congenitally blind human subjects, who are generally believed not to experience visual mental imagery, and are thus presumably rotating haptic or kinesthetic image
A. Michael Noll is an American engineer and actually a very early pioneer in digital computer art and 3D animation. He was one of the first people to propose that the digital computer might become a creative artistic medium. Most of his early work on digital art was done in FORTRAN.
Here is an example of Noll's early work - a three-dimensional projection of a rotating four-dimensional hypercube
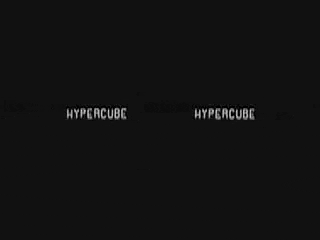